The 2022 Scientific Consensus Statement (SCS) brings together the latest scientific evidence to understand how land-based activities can influence water quality in the Great Barrier Reef, and how these influences can be managed to improve water quality outcomes for the Great Barrier Reef. The SCS is updated periodically and is used by policymakers as a foundational evidence-based document for making decisions about managing Great Barrier Reef water quality. It is one of several projects that provides supporting information for the design, delivery and implementation of the Australian and Queensland government’s Reef 2050 Water Quality Improvement Plan (WQIP). The WQIP defines objectives and targets related to water quality improvement, identifies spatial management priorities and describes actions for improving the quality of the water that enters the Great Barrier Reef from the adjacent catchment area.
The following list of Frequently Asked Questions (FAQs) provides brief non-technical answers that complement the 2022 SCS Question-level Evidence Syntheses by addressing topics that span more than one SCS Question or providing additional explanatory material for the SCS Conclusions. The following questions were selected based on policy and stakeholder interests, previous discussions with the Australian and Queensland government Contract Managers and FAQs from the 2017 SCS.
Status of the Great Barrier Reef
The Great Barrier Reef covers an area of 348,700 km² from the tip of Cape York to Bundaberg (including 24,094 km² of coral reefs, 35,679 km² of seagrass meadows, 2,188 km² of mangroves, 1,757 km² of salt flats and saltmarshes, and 15,556 km² of wetlands).
The current condition of the Great Barrier Reef varies among ecosystems and locations. Measurements from the Marine Monitoring Program show that the condition of Great Barrier Reef inshore coral reef ecosystems from the Wet Tropics to the Fitzroy region has declined marginally since 2017. In 2020 to 2021[1] inshore reefs were categorised overall as being in ‘Poor’ Condition (based on an index combining several indicators of coral health) with some differences between regions. On shallow midshelf and outer shelf reefs, measurements from the AIMS Long Term Monitoring Program show that hard coral cover has increased overall since 2017. Fast recovery has been recorded on midshelf and outer shelf reefs from Cooktown to Bundaberg following repeated mass coral bleaching events and/or crown-of-thorns starfish outbreaks between 2016 and 2019[2].
Inshore seagrass meadows monitored across the Great Barrier Reef through the Marine Monitoring Program declined from ‘Moderate’ abundance and resilience in 2017 to ‘Poor’ in 2020[1], and while overall condition improved in 2021 (to ‘Moderate’), there were continuing declines in the Fitzroy and Burnett Mary regions. These declines were primarily a consequence of above-average discharges from some rivers and disturbances from tropical cyclones. Mangroves and saltmarsh ecosystems are monitored less frequently and were considered stable and in ‘Good’ condition in 2018[3]. Wetlands are monitored as part of the Paddock to Reef program and are considered stable but in ‘Moderate’ condition[1], however, some wetland types have experienced significant regional losses historically.
[Note: the 2022 Scientific Consensus Statement included evidence up to December 2022, so the consequences of later impacts, such as the recent mass bleaching events in 2024 are not included. Refer to the 2024 Outlook Report for more up-to-date information on the health of Great Barrier Reef ecosystems.]
[1] Reef Water Quality Report Card 2020
[2] AIMS Long Term Monitoring Program
[3] Great Barrier Reef Outlook Report 2019
Further information: Q1.2/1.3/2.1
Threats to the Great Barrier Reef
Human-induced climate change is the overriding threat to the Great Barrier Reef, and the ability of ecosystems to recover from climate impacts and acute disturbances continues to be compromised by poor water quality.
The primary threat to the Great Barrier Reef is climate change. Studies over the last three decades confirm that the climate of the Great Barrier Reef is changing rapidly and in multiple ways, with some changes already significantly impacting Great Barrier Reef ecosystems, organisms and water quality. These impacts are occurring through rising temperature, increasing frequencies of marine heatwaves, increasing ocean acidification, sea level rise, and more extreme rainfall events. The impacts of climate change are likely to vary depending on the region. For example, in the southern Great Barrier Reef (south of Bowen), predictions indicate increasing frequency of droughts and drought-breaking floods, and in the northern Great Barrier Reef (north of Bowen), a potential reduction in the frequency but increasing intensity of tropical cyclones. There is consistent evidence that periods of extreme sea surface temperatures are causing mass coral bleaching and mortality. Thermal extremes also cause stress and damage to many other marine organisms including fishes, sponges, and seagrasses.
Poor water quality from the land-based delivery of fine sediments, nutrients, pesticides and other pollutants also poses a major threat to the Great Barrier Reef, especially for freshwater, coastal and inshore marine ecosystems. These environments provide critical ecosystem services across the Great Barrier Reef and maintain high tourism, aesthetic, spiritual, recreational and economic values. Poor water quality has direct impacts on Great Barrier Reef ecosystems (refer to FAQs 8 to 11 for additional information) but it can also reduce the ability of ecosystems to recover from climate-related disturbances. Other threats including tropical cyclones and storms, crown-of-thorns starfish outbreaks, direct use, and the combined effects of multiple stressors, can negatively affect the health of Great Barrier Reef ecosystems.
Further information: Q1.1, Q1.2/1.3/2.1, Q2.2, Q2.4
There is consistent evidence that climate change factors (including temperature and ocean acidification) and poor water quality (including elevated nutrients and sediments, reduced light and presence of pesticides) have combined effects on a variety of organisms in the Great Barrier Reef. In most cases, the outcome for the organism is worse under these combined stressors. For corals, the most harmful effects documented are the combined effects of climate change and herbicides. There is also evidence that climate change can interact with both nutrients and light reduction/sedimentation to cause additional stress to corals resulting in reduced growth, increased mortality, decreased photosynthesis, and reduced tolerance to higher temperatures. Several interactions between climate change and water quality have also been detected in seagrass ecosystems, with the possible exception of ocean acidification which can stimulate plant growth.
There is high confidence that improving water quality will to some extent ameliorate climate change impacts (‘buy time’) for coral reef and seagrass ecosystems, although the strength and length of this reprieve cannot yet be quantified. Improved water quality also indirectly benefits coral reef ecosystems by increasing the resilience of organisms and reducing recovery time following acute disturbances such as bleaching, crown-of-thorns starfish outbreaks and cyclones. These benefits will become increasingly important as climate pressures continue to grow.
The evidence confirms the urgency of meeting all Great Barrier Reef water quality targets[4] within the next ten years before climate impacts exceed the capacity for Great Barrier Reef ecosystems to recover.
Further information about the influence of poor water quality on the Great Barrier Reef and the primary sources of land-based pollutants are described in FAQ 8 to 12.
[4] The 2025 targets defined in the Reef 2050 Water Quality Improvement [currently under review] require a 25% reduction in the 2009 anthropogenic end-of-catchment fine sediment loads, 20% reduction of particulate nutrients, and a 60% reduction of dissolved inorganic nitrogen loads. The target for pesticides is to protect at least 99% of aquatic species at end-of-catchments by 2025.
Further information: Q2.4
The ocean absorbs about a quarter of the carbon dioxide (CO2) released into the atmosphere every year, so as atmospheric CO2 levels increase, so do the levels in the ocean. Absorbing greater amounts of carbon dioxide affects the chemical composition of seawater by lowering the pH level and making the ocean more acidic. Ocean acidification is increasingly recognised as an important pressure for coral reefs globally.
The increased acidity is of particular concern for organisms that use calcium carbonate to build shells or external skeletons, including hard corals and crustose coralline algae found on reefs. Ocean acidification can cause the dissolution of reef sediments and greater reef bioerosion both of which affect the reef’s structural framework, the proliferation of fleshy macroalgae, negative effects on coral recruitment, negative effects on crustose coralline algae (which provide a settlement platform for coral larvae), and a multitude of other adverse changes to the physiology, behaviour and ecology of tropical marine organisms and ecosystems. Some of these effects are similar in their direction to the effects of poor water quality, suggesting that water quality improvements may mitigate some of the effects of ocean acidification on inshore reefs.
Ocean acidification is affecting the whole Great Barrier Reef however some regions may be more vulnerable than others. The southern inshore reefs of the Great Barrier Reef are predicted to be more affected by warming temperatures than other areas, which can exacerbate the effects of ocean acidification. By 2030, the evidence consistently indicates that some reefs will already start experiencing critical acidification levels, diminishing reef accretion and reef recovery rates.
Further information: Q2.2
Crown-of-thorns starfish are coral eating starfish that occur naturally throughout the Indo-Pacific region. Densities of crown-of thorns starfish vary greatly both spatially and temporally, but when starfish are found in high numbers above naturally occurring levels (termed a population outbreak), they can cause considerable damage to reefs. Crown-of-thorns starfish outbreaks are one of the main causes of widespread declines in live coral cover on the Great Barrier Reef.
Following a big rainfall event, nutrients can run off the land into river systems which can lead to elevated nutrient levels in Great Barrier Reef waters. Elevated nutrient levels cause phytoplankton blooms that sometimes coincide with the larval stage of the crown-of thorns starfish. These blooms increase the amount of food available for larvae which can increase survival, growth, and development rates. Crown-of-thorns starfish outbreaks in the Great Barrier Reef start on midshelf reefs typically between Cairns and Lizard Island in an area called the ‘initiation area’. This overlaps with an area where nutrient-enriched river discharge enters the midshelf waters of the Great Barrier Reef on a regular basis. Although land-based nutrient runoff may contribute to outbreaks, other factors such as marine upwellings, life history traits including high fecundity, and the effect of fishing or removing predators that eat crown-of-thorns starfish may also be important. Combining evidence from these different factors can contribute to a more complete understanding about when, where and how population outbreaks will occur.
Further information: Q4.3
Land-based pollutants, including sediments, nutrients and pesticides, typically enter waterways when they run off the land during rainfall events. These materials can then be discharged into the Great Barrier Reef and carried in flood plumes. The influence of land-based pollutants, particularly fine sediments, nutrients and pesticides, is spatially and temporally variable between pollutants and locations, but it is most pronounced in freshwater, estuarine, coastal and inshore marine environments. Based on coral cores and other proxy records, the footprint of land-based runoff can be seen on the inner Great Barrier Reef shelf over 100 kilometres alongshore from the river mouth of influence.
Most sediment-laden flood plumes are confined to the inner shelf of the Great Barrier Reef, but during periods of large river discharge and low wind speeds, fine sediments originating from land and associated particulates can be carried further offshore to the middle shelf and even the outer shelf (particularly for the areas northwards of Bowen where these areas are closer to the coast). Reduced water quality can persist for up to six months due to frequent resuspension of this newly delivered sediment. The greatest impacts of fine sediments and particulate nutrients occur in the inshore central and southern Great Barrier Reef (Wet Tropics to Burnett Mary Natural Resource Management regions).
Nutrient concentrations originating from land-based activities follow a similar cross-shelf gradient with the highest concentrations found in estuaries and inshore waters, and lower values in midshelf and offshore waters. Peak concentrations are usually found during the wet season between Cooktown and Gladstone, adjacent to areas of more intensive catchment development and in waters influenced by river discharge. Marine processes such as upwelling, where nutrient-rich water moves from depth towards the surface, and nitrogen fixation can also influence nutrient variability in other areas of the Great Barrier Reef. Comparing the effects of different types of nutrients between and among regions is challenging due to limited spatial data.
Pesticides are ubiquitous across monitored Great Barrier Reef ecosystems including end of-catchment waterways, wetlands and in estuarine and nearshore marine habitats. Concentrations of pesticides decrease with greater distance from the site of application, and exposure of marine ecosystems to pesticides is closely linked to flood plume dispersal. Monitoring sites in the Mackay Whitsunday region and the Barratta Creek in the Burdekin region record consistently higher concentrations of pesticides and higher pesticide risk than other locations. These regions have intensive cropping activity which can involve the use of large amounts of pesticides, and experience smaller rainfall events than areas like the Wet Tropics. Lower rainfall means that there is less dilution of pesticides, so they are still highly concentrated when they reach aquatic ecosystems. Regarding other pollutants, metal concentrations are usually higher near more industrial and developed areas.
Overall, end of-catchment waterways, wetlands and marine ecosystems nearest to the mainland (including estuarine, coastal and inshore areas) are most exposed to chronic poor water quality associated with land-based runoff. While these areas generally represent a relatively small proportion of the Great Barrier Reef, they provide critical ecosystem services (such as habitat for many species, connectivity, carbon sequestration, etc.) and maintain high tourism, aesthetic, spiritual (including for Indigenous people), and recreational values (such as fishing, boating, diving, etc.).
While sediments and nutrients have always run off the land and entered river systems, there are multiple lines of evidence demonstrating that the volume of river discharge and loads of suspended sediment, dissolved and particulate nutrients (nitrogen and phosphorus), and pesticides have increased for most river basins of the Great Barrier Reef catchment area since the arrival of Europeans c. 1850. The evidence suggests that pollutant loads from the catchment area to the Great Barrier Reef have increased from pre-development loads by 1.4 to 5 times for fine sediments, and 1.5 to 3 times for dissolved inorganic nitrogen (with variations depending on basins). The increases in loads have largely occurred because catchments have been modified for the major land uses of livestock grazing (73% of catchment area), irrigated and dryland cropping (2.8%), sugarcane (1.2%), horticulture and bananas (0.2%), urban development (0.7%) and mining (0.3%), in combination with long term climate variability.
There is a complex interaction between natural and anthropogenic sediment delivery and processing in the Great Barrier Reef. It is recognised that the dominant influence on water clarity in the inner shelf of the Great Barrier Reef is wave-driven resuspension followed by tidal resuspension. However, the volume and timing of the delivery of land-derived sediment to the Great Barrier Reef has changed since the arrival of Europeans, increasing the area of the inner and middle shelfs affected by reduced light from lower water clarity. This is supported by multiple lines of evidence from turbidity loggers, remote sensing and modelling which show that higher and longer periods of lower water clarity and corresponding longer periods of reduced light in the water column in certain areas of the inner and middle shelfs of the Great Barrier Reef coincide with years of increased river discharge and associated sediment loads.
Several studies also show that most river-exported sediment is deposited and retained within river floodplains, river estuaries, close to river mouths and within the eastern sections of north-facing bays. A proportion of the fine sediment (<20 µm) load can be carried within flood plumes to the inner and middle shelfs. This fine material settles as a ‘fluffy layer’ on the seafloor and is more easily resuspended under less-energetic wave events than the existing compacted sediment. This process results in longer periods of reduced light in sections of the inner and middle shelfs in the years of above average discharge and sediment loads. For example, monitoring data shows that reduced water clarity caused by sediment-laden flood plumes can persist for up to six months due to frequent resuspension of newly delivered sediment.
Peak concentrations of dissolved nutrients are usually found during the wet season (typically December to May) in the central and southern Great Barrier Reef (approximately from Cooktown to Gladstone) adjacent to areas of more intensive catchment development and in waters influenced by river discharge. However, offshore and midshelf variations in nutrient concentrations in surface waters can also be associated with upwelling events (which sometimes bring dissolved inorganic nitrogen and phosphorus from deeper water to the surface) and blooms of the algae Trichodesmium (which fix atmospheric nitrogen). Although river discharges are still considered to be the dominant driver of dissolved nutrients in inshore waters, more research is needed to fully understand the contribution of upwelling versus anthropogenic inputs on midshelf areas of the Great Barrier Reef.
Sediments in water are measured as ‘total suspended solids’ or ‘total suspended sediment’, and are characterised by different particle sizes, for example, clay, silt and sand. It is the fine fraction (silt and clay) that is of greatest concern to marine ecosystem health due to the ecological impacts and because this fine material can be transported long distances from the river mouth.
Increases in the loads of fine sediments (and associated particulate nutrients) affect the quantity and quality of light reaching coral reefs, seagrass and other benthic organisms. Changes to the quantity and quality of light in the water column can negatively affect organisms that depend on adequate light levels for growth and energy supplies (e.g. corals and seagrasses) and can influence the behaviour and condition of animals including fish, particularly in inshore areas. Changing light conditions have resulted in persistent impacts on reef composition (including changes in the abundance and diversity of species at different depths, and increases in macroalgal growth), and variability in the distribution, abundance and composition of seagrass meadows. These direct effects can result in indirect effects on other dependent organisms such as fish and dugong.
Sedimentation, the settling of sediments and particulate nutrients onto surfaces, can also have negative effects on a variety of organisms including corals, sponges and seagrasses, by causing tissue damage, reducing growth rates and altering microbial communities. Importantly, settled and suspended particulate matter can impede recovery from other disturbance events (such as heatwaves and cyclones) and affect the early life stages of corals and fishes.
Further information: Q3.2
Excessive amounts of dissolved nutrients have an impact on Great Barrier Reef ecosystems. In coral reefs, the most severe impacts of dissolved nutrients are indirect, for example by promoting increased fleshy macroalgal abundance and growth on inshore reefs. Macroalgae can outcompete corals, reduce the ability of new corals to settle, negatively affect coral growth and reduce coral cover. Excess nutrients may also contribute to coral-eating crown-of-thorns starfish outbreaks, as they can promote phytoplankton blooms, which creates more food for larvae, potentially enhancing their survival, growth, and development rates. However, other factors such as marine upwellings, life history traits including high fecundity, and the effect of predator removal through fishing may also be important drivers of crown-of-thorns starfish outbreaks.
Direct effects of elevated nutrients on Great Barrier Reef ecosystems include reduced coral calcification (the ability of corals to build their skeletons and grow), negative impacts on coral reproduction, and potentially making corals more susceptible to bleaching at lower temperatures.
Comparing the effects of dissolved inorganic nutrients between and among regions is challenging due to limited spatial data. Reef communities from the Mackay Whitsunday Natural Resource Management region, and to some extent the Burdekin region have been relatively well studied, but there is a significant lack of information from other areas of the Great Barrier Reef.
There is no clear evidence of direct negative impacts of increased nutrients on seagrass ecosystems. Elevated nutrients may be beneficial for mangrove growth, but when combined with climate stressors such as drought (low rainfall and low humidity) can cause mangrove decline. There is limited evidence of the impact of dissolved nutrients on Great Barrier Reef wetlands.
Further information: Q4.2
Pesticides are present across all monitored Great Barrier Reef ecosystems, including end-of-catchment waterways, wetlands and in estuarine and nearshore marine habitats. Their presence in the offshore marine environment is largely unknown. Exposure of marine ecosystems to pesticides is closely linked to flood plume dispersal.
Pesticides, including herbicides, insecticides and fungicides, are designed to control agricultural pest species, and virtually all tested pesticides are potentially harmful to aquatic species in the Great Barrier Reef. Pesticides pose an ecological risk if they occur at concentrations greater than a relevant toxicity threshold or guideline value. For example, herbicides impact all photosynthetic (i.e. use light as an energy source) marine organisms of the Great Barrier Reef that have been tested, including corals and seagrass. Other simultaneous pressures, including heatwaves and variation in light, can increase the sensitivity of Great Barrier Reef species to pesticides, indicating that guideline values applied under some conditions in the field are likely to underestimate the risk to aquatic ecosystems.
Monitored pesticides that contribute most to risk in all Great Barrier Reef ecosystems examined include atrazine, diuron, imidacloprid and metolachlor. Monitoring in the Mackay Whitsunday region and Barratta Creek in the Burdekin region recorded consistently higher concentrations of pesticides and higher risk than other locations. These regions have intense cropping activity which can involve the use of large amounts of pesticides, and experience smaller rainfall events than areas like the Wet Tropics. Lower rainfall means that there is less dilution of pesticides, so they are still highly concentrated when they reach aquatic ecosystems.
Further information: Q5.1
Other pollutants present in the Great Barrier Reef include metals, persistent organic pollutants, per- and poly-fluoroalkyl substances, plastics, pharmaceutical, veterinary and personal health care products, coal dust, and sunscreens. There is insufficient monitoring data to determine the spatial range, concentrations, temporal trends and the risk that most of these pollutants pose to Great Barrier Reef ecosystems.
Metal concentrations in water and sediments are higher in more industrial and developed coastal environments compared to less developed catchments and offshore areas. Concentrations of metals in water above national water quality guideline values are rarely documented but have been recorded in some studies including copper (associated with legacy mining in the Fitzroy basin), mercury (associated with sugarcane in the Tully catchment) and aluminium (from acid sulfate soils in Trinity Bay, Cairns). Organisms found inshore (e.g. seagrass, algae, turtles, corals) have higher concentrations of metals in their tissues than those found offshore. Although ecotoxicological studies indicate that the ecological risks from metals in the Great Barrier Reef are relatively low and constrained to a few small locations, the combined risks from mixtures have not been assessed.
Persistent organic pollutants are associated with industry, oil spills, coal, and urbanisation. They are detectable in Great Barrier Reef sediments (generally below guideline values, with exceptions for example after an oil spill) and in animals and plants, and from the limited data available, decrease across an inshore to offshore gradient. Persistent organic pollutants can affect fish physiology and behaviour, coral reproduction and trophic food webs.
Plastics, including microplastics and fibres, are extensively distributed in coastal and marine environments. Coastal sites are influenced by surrounding land use, river and stormwater inputs, while offshore sites are mostly influenced by recreational activities, tourism, commercial shipping and fishing. Plastics have been recorded in zooplankton, crustaceans, fishes, birds and turtles from the Great Barrier Reef, with ecological risks varying across species, feeding behaviour and life stages.
A more cohesive and coordinated approach to examine the interaction of multiple pollutants and stressors, including climate change, is still required.
Further information: Q6.1
Sources of pollutants from the catchment to the reef
Pollutant loads from the catchment area to the Great Barrier Reef have increased from pre-development loads by 1.4 to 5 times for fine sediments, and 1.5 to 3 times for dissolved inorganic nitrogen (with variations depending on basins). The increases in loads have largely occurred because of land use changes, in combination with long term climate variability.
The Burdekin and Fitzroy basins are the largest exporters of total fine sediment and particulate nutrients to the Great Barrier Reef, each exporting an annual average load of over 3,000 kilotonnes and 1,300 kilotonnes of fine sediment per year, respectively, and more than 3,000 tonnes of particulate nitrogen per year. It is estimated that grazing lands contribute 60% of the total fine sediment load from 73% of the Great Barrier Reef catchment area, sugarcane contributes 10% from 1.2% of the area, irrigated and dryland cropping contribute 4% from 2.8% of the area, urban contributes 2% from 0.7% of the area, and bananas and horticulture contribute 1% from 0.2% of the area. Other land uses such as nature conservation and forestry collectively contribute 23% of the total fine sediment load from approximately 22.1% of the Great Barrier Reef catchment area, but this is natural, not anthropogenic export. The main drivers of anthropogenic sediment and particulate nutrient exports are overgrazing, land clearing, tillage and other soil disturbances. Modelling and observations show that about half of the sediment exported comes from gully erosion, with hillslope and streambank erosion accounting almost equally for the other half. Much of the export comes from a relatively small area. For example, in the Bowen catchment, 30% of the sediment from gully erosion comes from just 2% of the gullies.
Basins dominated by sugarcane make the greatest contribution to dissolved inorganic nitrogen exports (42% of total), including those in the Wet Tropics, Burdekin and Mackay Whitsunday Natural Resource Management regions. Grazing lands contribute 22%, and although other land uses such as urban and bananas and horticulture contribute smaller amounts, they can be locally important. Increased erosion from grazing and other land uses can contribute to nutrient export. The primary drivers of anthropogenic nitrogen and phosphorus export are fertiliser application, changed catchment hydrology and erosion.
Sugarcane is the main source of pesticides, with other agricultural and urban sources also contributing. Pesticide export is influenced by the timing and rate of application, rainfall, irrigation regimes, and pesticide and soil properties. Sites in the Mackay Whitsunday region and Barratta Creek in the Burdekin region, that have intense cropping consistently record higher concentrations of pesticides and higher risk than other monitored locations.
Particulate nutrients, including particulate nitrogen and particulate phosphorus, generally have the same biophysical drivers as fine sediments, including vegetation degradation, surface disturbance from cattle trampling, feral animals, tillage in cropping areas, historical surface mining, unsealed roads and construction earthworks.
Generally, particulate nutrients are transported from the catchment to the marine environment attached to soil and therefore have similar impacts on ecosystems as fine sediment. Recent studies on particulate nutrients in flood plumes and river estuaries highlight the potential for rapid transformation of particulate nutrients to bioavailable forms (dissolved nutrient forms that are readily consumed by algae) within coastal areas. Frequent resuspension of sediments within estuaries and the coastal zone helps promote rapid cycling of particulate nutrients which are largely mineralised through microbial communities. The potential impacts are therefore the same as for dissolved nutrients (see FAQ 9) and can include increased growth of fleshy macroalgae on inshore reefs, increased phytoplankton blooms, and other direct impacts on corals such as reduced calcification, reduced reproductive capacity, and increased susceptibility to bleaching.
Bioavailability depends on sediment characteristics such as soil type, land use and sediment source (surface or subsurface). However, additional research is still needed to quantify the generation of bioavailable nutrients from soil erosion and the timeframes of nutrient transformation. The few studies investigating these processes have been conducted in the Burdekin and Wet Tropics regions, and very little research into nutrient transformation has been conducted in other regions. One area that requires further study is the source of land-based dissolved inorganic nitrogen in the Fitzroy region, as this region is dominated by grazing lands, which indicates that soil erosion could be a large source of nutrients.
About half of the sediment exported comes from gully erosion, with hillslope and streambank erosion accounting almost equally for the other half. The most important primary biophysical drivers of anthropogenic sediment and particulate nutrient exports to the Great Barrier Reef include vegetation degradation (land/tree clearing, low ground cover, and changes in structure and function of grass species including a shift to non-native pastures, typically in response to long term grazing pressure), surface disturbance from cattle trampling, feral animals, tillage in cropping areas, historical surface mining, unsealed roads and construction earthworks. All of these drivers make it easier for soil and sediment to be moved around the landscape and transported to the Great Barrier Reef. While the importance of these drivers varies between erosion types, in different locations and at different times, gully erosion is strongly driven by surface disturbance and vegetation degradation / low ground cover, and streambank erosion is accelerated by removal or degradation of riparian vegetation and grazing pressure. Degraded soil conditions and the capacity of roads, tracks and fence lines to concentrate runoff are also important drivers in some locations. Rainfall is a natural driver which determines the timing of sediment exports.
Areas downstream of dams and closer to the coast lead to higher rates of sediment and particulate nutrient delivery to the coast, therefore the location of sites for targeting management is important when estimating management outcomes and translating load reductions between the site, and what is delivered to the end-of-catchment.
The evidence used in the 2022 Scientific Consensus Statement draws on multiple lines of evidence, including experimental and observational data, monitoring, modelling, remote sensing observations, as well as theoretical or conceptual evidence. Due to the cost, logistical challenges, and long timeframes involved in measuring improvements in water quality following land management change, a common approach to predicting the effectiveness of practice change is to use modelling. Modelling studies are often conducted because i) there is insufficient time and money available to measure the response of land management practice; ii) there are large areas where there is no monitoring data available; iii) modelling provides a consistent approach across large spatial scales; and iv) modelling allows ‘what-if’ scenarios to be run to support investment decisions.
The Great Barrier Reef catchment models use measured changes in land management and well-documented and accepted methods and assumptions. Long-term water quality monitoring data is used to validate and improve the models, continuously improving confidence in the estimates of water quality over time[5].
[5] https://www.reefplan.qld.gov.au/tracking-progress/reef-report-card/2021-22/faqs#toc-16
Further information: Q3.5, Reef Water Quality Report Cards
Monitoring and evaluation of projects and programs that involve management actions to improve coastal and marine water quality are essential to assess environmental, social and management change, track progress towards program objectives and targets, and inform and improve current and future decision making. Monitoring is a critical element that involves the collection of data and information before, during and after implementation. Successful evaluation involves the systematic assessment of a project or program’s design, its implementation, and outcomes to determine whether original objectives were achieved, identify lessons learned, deliver learning and demonstrate accountability. Coastal and marine water quality monitoring and evaluation programs that have successfully driven positive change from management around the world include those that adopt the system drivers, pressures, state, impact and responses (typically shortened to DPSIR) framework, recognise ecosystem services and marine natural capital, adopt multi-disciplinary frameworks, report on the interactions between environmental and human health, and support connections to people through the use of citizen science.
The Reef 2050 Integrated Monitoring and Reporting Program and the Paddock to Reef Integrated Monitoring, Modelling and Reporting programs are among the most comprehensive and integrated catchment to reef monitoring programs in the world. These programs recognise links between drivers, pressures and state through the reporting of environmental, social and economic indicators. Potential improvements could include greater recognition and quantification of complex social, cultural, economic and environmental values and their interconnections, extension of existing multidisciplinary frameworks to incorporate human health, and enhanced community engagement including direct participation in monitoring programs. A holistic ecosystem approach to Great Barrier Reef water quality management in the context of other major drivers such as climate change could also help to enhance the value of existing monitoring and evaluation programs.
Further information: Q8.2
Management actions to improve Great Barrier Reef water quality
Good water quality is critical for healthy and resilient ecosystems and supports recovery from disturbances such as mass bleaching and extreme weather events.
Poor water quality (e.g. elevated nutrients, increased sediment and pesticides) and climate change (e.g. increased temperature and ocean acidification) both put pressure on organisms and ecosystems. Organisms and ecosystems already stressed by poor water quality are less able to cope with climate change impacts and the evidence suggests that when these stressors are combined, the outcomes are often worse. Improving water quality therefore is a localised action that can alleviate some of the pressure facing the Great Barrier Reef. Improved water quality can indirectly benefit ecosystems by increasing the resilience of organisms and reducing recovery time following acute disturbances such as bleaching, crown-of-thorns starfish outbreaks and cyclones. Resilience will become increasingly important as climate pressures continue to grow.
The evidence within the 2022 Scientific Consensus Statement confirms the urgency of meeting all Great Barrier Reef water quality targets within the next 10 years, before climate change impacts exceed the capacity for Great Barrier Reef ecosystems to recover.
The most effective management practices for reducing fine sediment and particulate nutrient export from the Great Barrier Reef catchment area vary between land uses and erosion types, either hillslope, gully or streambank erosion (see FAQs 19 to 21). Common practices across land uses include maintaining or reintroducing vegetation into landscapes (including pasture management and vegetation buffers on waterways), reducing the hydrological connectivity of flow pathways (via management of roads, drains, gullies, etc.), and other practices that minimise soil runoff (such as green cane trash blanketing, zero/minimum tillage and controlled traffic farming).
Information on the longer-term effectiveness, costs and production outcomes of sediment management actions for all land uses and rehabilitation activities, including maintenance requirements for gully and streambank treatments requires further study. In addition, adoption of a consistent, peer reviewed approach for assessing the cost-effectiveness of fine sediment and particulate nutrient management actions is needed.
FAQs 19, 20 and 21 provide further information on management options for each erosion type.
Hillslope erosion, which is estimated to contribute to 22% of the total export of fine sediments to the Great Barrier Reef, increases sharply as ground cover declines below 30–50% in agricultural lands. This is because low ground cover exposes soil to erosion following rainfall and enables runoff to travel more easily through landscapes compared to more complex, vegetated habitats which can slow flow and promote deposition within channels, floodplains, and wetlands. The most effective practices for reducing hillslope erosion from agricultural lands include the use of moderate and adaptive grazing stocking rates, maintenance of at least 40% ground cover (but preferably >70%), regular periods of strategic rest from grazing (especially in the early wet season), cattle exclusion from fragile land-types, maintaining or reintroducing vegetation into landscapes (including pasture management and vegetation buffers), management of sediment delivery pathways within catchments (e.g. via management of roads, drains and gullies), and other practices that minimise soil runoff (such as green cane trash blanketing, zero/minimum tillage and controlled traffic farming). There is high variability in the cost-effectiveness of practices at the farm/project scale which is driven by several factors including a wide range of different practices and economic returns, location within the landscape and factors relating to sediment mobilisation and delivery.
There are few studies related to the effectiveness of sediment management in the Great Barrier Reef catchment area for bananas/horticulture, urban and roads compared to grazing, sugarcane and cropping.
In urban areas, effective practices for sediment management aim to reduce runoff and are linked to improving filtration, hydrological connectivity of impervious surfaces, and greater runoff retention times. Combining treatments into treatment trains (which are a set of hydrologically linked treatments) is more effective than single treatments. For most technologies, there is limited reliable field data and few studies that provided any cost or cost-effectiveness data.
For roads, effective practices for sediment management include revegetation on roadsides, engineering drainage design, and specific erosion control measures such as the use of erosion control blankets, geotextiles, silt fences and compost/mulch. For unsealed roads, road surface gravelling can also be effective, however, this varies with gravel type. While there are guidelines for road management relevant to construction and maintenance, there are limited studies of the water quality and cost outcomes of treatments. The distribution, density and water quality impacts of roads are not documented.
Further information: Q3.5
It is estimated that around half of the total fine sediment load delivered to the Great Barrier Reef is from gully erosion. The large-scale remediation of alluvial (river associated) gullies has been demonstrated to be an effective strategy to significantly reduce fine sediment load delivered to the Great Barrier Reef. Gully remediation treatments can include major earth works and reshaping, soil treatment, installation of rock chute structures, earth bunds and water points, fencing and revegetation. A combination of these treatments can achieve over 90% fine sediment reduction within one to two years. Ongoing maintenance (including livestock exclusion) of the remediation sites is required to retain these benefits. Alluvial gully treatments typically have relatively short response times in terms of fine sediment reductions and can be treated at large scales, resulting in logistical efficiencies. Of relevance to management prioritisation, the available studies (in the Bowen catchment in the Burdekin basin) also indicate that a small number of gullies (~2% of the total number) contribute a substantial proportion of the sediment yield (30%), highlighting the need for targeting the high yielding gullies as a means of efficiently reducing fine sediment exports to the Great Barrier Reef. Large-scale remediation of high sediment yielding gullies has been demonstrated to be cost-effective and is 26–60 times more cost-effective in achieving the same cumulative fine sediment reduction than lower-cost options for lower-yielding gullies. The limited available peer reviewed evidence shows that the remediation of hillslope gullies using options such as low-cost check dams in gully floors used to slow water flow, and fencing used to keep out large animals, is less cost-effective compared to remediation of large high-yielding gullies.
Further information: Q3.6
It is estimated that around a quarter of the total fine sediment load delivered to the Great Barrier Reef is from streambank erosion. Streambank rehabilitation treatments include interventions to increase riparian vegetation, either directly through planting, or indirectly through the removal of disturbance pressures such as grazing to encourage natural colonisation, and in some cases bank reprofiling and stabilisation, which enables subsequent revegetation via planting and/or natural colonisation. Rehabilitation works are difficult to evaluate due to limited measurement of treatment effectiveness in the Great Barrier Reef catchment area, but studies have shown that bank erosion generally occurs at lower rates on vegetated streambanks than non-vegetated streambanks. This is because a lack of vegetation enables runoff to move more easily through landscapes compared to more complex, vegetated habitats which can slow flow and promote deposition. Whole-of-system approaches that seek to maximise recovery of riparian vegetation at the river reach to network scale are required. While streambank rehabilitation will assist in reducing sediment export in the Great Barrier Reef catchment area, estimates of return on investment are poorly understood.
Further information: Q3.6
Tree clearing and vegetation loss from cattle grazing and cropping has been identified as a major driver of anthropogenic sediment and particulate nutrient exports to the Great Barrier Reef. These drivers are continuing and appear to be increasing sediment exports over time, especially where they occur in areas prone to, or experiencing, gully and streambank erosion. Protecting vegetation in these areas is a priority.
Actions that reverse vegetation degradation and prevent surface disturbance can reduce exports by making it harder for runoff to move across landscapes, especially when actions are targeted within gully networks and riparian zones. The erosion rate of gully walls is inversely related to vegetation cover, so it can be expected that gully wall revegetation will reduce sediment export.
Climate change is projected to increase the magnitude of large floods, cause more severe droughts and alter fire regimes, all of which may lead to further vegetation degradation and drive gully and streambank erosion processes to increase future export volumes and concentrations. Therefore, the need for vegetation protection in areas of sediment supply is increasingly important for reducing sediment runoff to the Great Barrier Reef.
Further information: Q3.4
Catchment restoration involves actions that can help address drivers of poor water quality. In the Great Barrier Reef catchment area, efforts have focused on gully, streambank and wetland restoration.
Gully remediation treatments can include major earth works and reshaping, soil treatment, installation of rock chute structures, earth bunds and water points, fencing and revegetation. Remediation of large alluvial gullies has been demonstrated to be a highly effective strategy to significantly reduce fine sediment loads delivered to the Great Barrier Reef, achieving over 90% fine sediment reductions within one to two years when a combination of treatments is applied. Ongoing maintenance (including livestock exclusion) of the remediation sites is required to retain these benefits. Few studies focus on measuring the water quality benefits of streambank restoration, however, it is evident that bank erosion generally occurs at lower rates on vegetated streambanks than non-vegetated streambanks.
Streambank rehabilitation treatments include interventions to increase riparian vegetation, either directly through planting, or indirectly through the removal of disturbance pressures such as grazing to encourage natural colonisation, and in some cases bank reprofiling and stabilisation, which enables subsequent revegetation via planting and/or natural colonisation. There is a need to refocus efforts from site-scale management to whole-of-system approaches that seek to maximise recovery of riparian vegetation at the river reach to network scale, rather than focusing on individual erosion sites.
Extensive wetland areas of the Great Barrier Reef, particularly across floodplains, have been lost over time, while others have become increasingly degraded. Wetlands have the potential to retain and process pollutants such as sediments, nutrients and pesticides, and therefore restoration of wetlands by improving or restoring hydrological connections and revegetation has the potential to provide water quality benefits. However, without a long-term plan of maintenance, and clear definition of restoration goals, restoration project sites have a high risk of returning to a degraded state, reducing the services they provide.
Land management and remediation actions are most cost-effective when targeted at hotspots of pollutant export, however, further work is required to understand the return on investment for these restoration actions in the Great Barrier Reef context.
Riparian areas are defined as any area within 50 metres of a (mapped) stream or riverine wetland[6]. Removal or degradation of riparian vegetation can accelerate streambank erosion, which contributes to 24% of the total load of sediments exported to the Great Barrier Reef.
Riparian areas can be rehabilitated either directly through planting, or indirectly through the removal of disturbance pressures such as grazing to encourage natural colonisation. In some cases, bank reprofiling and stabilisation, which enables subsequent riparian revegetation via planting and/or natural colonisation can be an appropriate intervention technique. There is a need to refocus efforts from site-scale management to whole-of-system approaches that seek to maximise recovery of riparian vegetation at the river reach to network scale, rather than focusing on individual erosion sites.
[6] https://www.reefplan.qld.gov.au/tracking-progress/reef-report-card/2021-22/faqs#toc-20
Wetlands are vital for sustaining the health and resilience of the Great Barrier Reef, and cover ~4% of its catchment (including artificial/highly modified, lacustrine, palustrine or vegetated swamps, riverine and estuarine). Natural and near natural wetlands provide many benefits to society and the environment including regulating ecosystem services such as improved water quality and carbon sequestration, supporting services such as nutrient cycling and provision of habitat, cultural services such as aesthetics and recreation and provisioning services including food, water and other resources.
There are few studies from the Great Barrier Reef that measure or model the efficacy or cost of wetlands as a water quality improvement tool. Global evidence shows that tropical wetland systems can retain, process and, at times, export nutrients, sediments, and pesticides with a wide-ranging capacity for pollutant retention. Natural and near natural wetlands are typically more effective at nutrient (certain forms) and pesticide removal than constructed or restored wetlands. Sediment is often retained in wetlands but can be remobilised in large flow events, for example after periods of heavy rainfall.
Critical factors for optimising the efficacy of water quality improvement in wetlands include: the presence and maintenance of vegetation communities; hydrological characteristics including the wetland size relative to the contributing catchment area, flow rate, loss pathways and water residence times; and the type, form and input concentration of the targeted pollutant. Available evidence indicates that the costs of treatment wetlands are highly variable. These factors, and the need for ongoing maintenance, need to be carefully considered when planning for the use of wetland systems as a water quality improvement tool in the landscape.
The historical loss of natural wetlands, particularly in floodplains and the degradation of those remaining are important considerations for maximising the efficacy of water quality improvements and determining future protection and management opportunities for Great Barrier Reef wetlands.
Knowledge gaps still exist around the role that wetlands can play as both an ecosystem asset and a regulating mechanism.
Nitrogen and phosphorus are the main nutrients that can impact Great Barrer Reef water quality and are linked to land management. The most effective and profitable management practice for reducing dissolved nitrogen exports from the Great Barrier Reef catchment area is reducing nitrogen fertiliser applications to industry recommended rates, with consistent results across different land uses, climates, and management contexts. Further rate reductions give consistent water quality benefits, but productivity and profitability vary. In sugarcane, other management practices to consider include the use of Enhanced Efficiency Fertiliser, mill mud application, improved irrigation, crop residue management, improved farming systems (such as growing legumes in between sugarcane crops) and burying fertiliser. For many of these management practices, the effectiveness and profitability are not clearly demonstrated and, for some, varies depending on climate, soil and seasonal characteristics. The effectiveness and profitability of practices for reducing dissolved phosphorus exports are less clear, as is the situation for crops other than sugarcane.
For urban and non-agricultural land uses, measures that include vegetation or biological components such as constructed wetlands, biofilters, algal ponds and existing riparian zones have considerable potential for removal of diffuse runoff nutrients and may also be important for management of wastewaters. Improvements in technologies for wastewater management also show that systems such as membrane filtration and chemical addition are likely to perform well. Other controls such as policy, planning, regulation and compliance appear to work best when applied as part of an integrated approach, and recycling and reuse show considerable potential.
Tropical wetland systems can also retain and process nutrients with a wide-ranging capacity for pollutant retention depending on the wetland type. Local evidence indicates that constructed or treatment wetlands can be most effective in processing nutrients however the results are highly variable. There are few studies that comprehensively measure or model the efficacy of wetland or costs of water quality improvement in the Great Barrier Reef catchment area.
The most effective management practices for reducing pesticide risk from the Great Barrier Reef catchment area vary between land uses. Practices that reduce pesticide risk from agricultural land uses include reducing the total amount of pesticide applied through lower application rates (within label recommendations), improving application methods, timing of application in relation to weather risk periods, switching to pesticide products with lower environmental risk and reducing soil erosion through retaining cover, controlled traffic and improved irrigation management for pesticides with greater soil sorption. These findings have remained relatively consistent through time, and across climatic regimes and farming systems of the Great Barrier Reef catchments.
The emergence of significant pesticide resistance across multiple industries has started to cause considerable changes in pesticide use and other alternative pest control measures.
A range of non-chemical pesticide control measures (integrated pest management, cultural controls that modify the pest’s growing environment) hold considerable potential for reducing reliance on chemical control measures, but most are yet to be trialled in the Great Barrier Reef catchment area with respect to long-term pesticide use reductions, efficacy and economic outcomes.
For non-agricultural lands, pesticide management options largely rely on controls such as regulations, and improved wastewater treatment processes (membrane bioreactors, reverse osmosis).
Further information: Q5.3
Co-benefits occur where a specific land management practice implemented to improve water quality for the Great Barrier Reef has additional positive on-farm secondary impacts such as improving economic and production outcomes, reducing carbon emissions, increasing biodiversity or improving soil health.
As an emerging topic of interest in the Great Barrier Reef catchment area, the information available to fully understand the co-benefits that can occur through management practices in grazing, sugarcane, bananas, horticulture and cropping (primarily grains) land uses to improve water quality is currently limited, but the available literature clearly indicates that there is potential for significant environmental and social co-benefits in some settings. For example, reducing grazing pressure and changing the timing of grazing can lead to increased vegetation cover, particularly of perennial grasses, which can improve water infiltration and reduce runoff, leading to improved soil health, higher levels of soil carbon and greater biodiversity. In sugarcane and grain cropping systems, nitrogen management strategies (type, timing, and quantity applied) implemented to reduce the amount of nitrogen entering the Great Barrier Reef can also reduce greenhouse gas emissions, particularly nitrous oxide. The use of break-crops, rotations, controlled traffic farming and trash blanketing, all of which are used to reduce runoff and erosion, also has demonstrated benefits for soil health.
Co-benefits associated with management practices to improve water quality can be complex and require careful planning and design. Contextual and site factors, the specific design and implementation of the management action and program design can influence the extent, magnitude and duration of a co-benefit.
The restoration of wetlands to enhance water quality benefits can also bring additional benefits to society and the environment including carbon sequestration, nutrient cycling, provision of habitat, cultural services such as aesthetics and recreation and provisioning services including food, water and other resources. However, without a long-term plan of maintenance, and clear definition of restoration goals, restoration project sites have a high risk of returning to a degraded state, reducing the services they provide.
Overall, expanded research effort and more consistent methods are urgently needed to adequately assess the co-benefits of management solutions across different landscape and climate conditions in the Great Barrier Reef catchment area.
The 2022 Scientific Consensus Statement
The development of the 2022 Scientific Consensus Statement has involved almost 200 experts, researchers, scientists, policy and management teams, and other stakeholders and groups from Australia and overseas.
C2O Consulting was appointed by the Australian and Queensland governments as an independent organisation to lead and coordinate the delivery of the 2022 Scientific Consensus Statement. The team at C2O Consulting has many years of experience working on the water quality of the Great Barrier Reef and its catchments and has been involved in the coordination and production of the Scientific Consensus Statement since 2008.
Groups and/or individuals involved in the development of the 2022 Scientific Consensus Statement included:
- Australia’s Chief Scientist
- Queensland Chief Scientist
- Probity Advisor
- Scientific Consensus Statement Coordination Team (C2O Consulting)
- Evidence Synthesis expert (Evidentiary)
- Methods Working Group
- Reef Water Quality Independent Science Panel (ISP)
- Reef 2050 Independent Expert Panel (IEP)
- Lead Authors & contributors (78 in total)
- Editorial Board (1 Editor-in-Chief, 6 Editors)
- Peer reviewers (69 in total)
- Consensus Process Working Group
- Stakeholders
- Reef 2050 Executive Steering Committee
- Australian and Queensland Government Contract Managers
A complete list of everyone involved in the 2022 Scientific Consensus Statement is available within the Summary document (here).
The 2022 Scientific Consensus Statement was developed between 2022 and 2024, and it was officially launched in August 2024.
The 2022 Scientific Consensus Statement adopted a formal evidence review and synthesis method to address 30 priority questions that were developed in consultation with scientific experts, policy and management teams and other key stakeholders including representatives from agricultural, tourism, conservation and research organisations, and Traditional Owner groups[7]. The evidence typically covers the period from 1990 until December 2022 (hence the name, ‘2022 Scientific Consensus Statement), with a few exceptions for articles that were published in early 2023 but were already approved or known by the author teams by the end of 2022 when the searches were being conducted.
The comprehensiveness of the processes adopted for question setting, author selection, the synthesis of the evidence, peer review and reaching scientific consensus have extended the amount of time required to deliver this iteration of the Scientific Consensus Statement. The processes put in place provide a strong foundation for future updates of the scientific evidence to support the Reef 2050 Water Quality Improvement Plan.
[7]Additional information on question setting, synthesis methods and individual outputs is available from the 2022 Scientific Consensus Statement website.
In the lead up to the 2022 iteration of the Scientific Consensus Statement, the Australian and Queensland governments sought expert advice and stakeholder feedback on how the process to develop the Scientific Consensus Statement could be improved. Of key importance, it was clearly identified that the process needed be more transparent to help build greater confidence and trust in the findings. As a result, several enhancements were made to the way that the 2022 Scientific Consensus Statement was developed, designed, and delivered compared to earlier iterations. Refinements include:
- Additional steps to ensure higher levels of independence from end users in the coordination of the project, synthesis of the evidence and review of the outputs.
- Development of a set of guiding principles to underpin the design, delivery and implementation of all aspects of the 2022 Scientific Consensus Statement.
- Obtained probity advice to manage real or perceived conflicts of interest throughout the process.
- Greater transparency about the approach used for each major step of the development process.
- Extensive consultation with policy, management, science and stakeholder representatives to identify and prioritise a series of specific questions (rather than broad chapters).
- Establishment of a transparent author selection process through broad calls for expressions of interest.
- The use of formal best practice evidence-based synthesis methods that supported transparency in the way that evidence was identified and synthesised, minimised bias and could demonstrate levels of confidence in the evidence.
- Increased rigour around the peer review process including the establishment of an independent Editorial Board to design and manage the process.
- Adoption of formal methods for reaching scientific consensus involving a large number of experts to develop the Conclusions and Summary.
- Ensured that the Summary and Conclusions documents were clearly linked to, and supported by, the underlying evidence base.
- Incorporation of an additional layer of assurance and oversight by Australia’s Chief Scientist to ensure that the processes to develop the 2022 Scientific Consensus Statement were transparent, robust and credible.
Further information: 2022 Scientific Consensus Statement Process and Assurance Statement from Australia’s Chief Scientist
Yes, all of the outputs of the 2022 Scientific Consensus Statement were independently reviewed. A formal independent Editorial Board (with one Editor-in-Chief and six Editors) was established to run the peer review process for the three primary outputs of the 2022 Scientific Consensus Statement, specifically, the 30 syntheses of evidence, Summary document and Conclusions.
The formal peer review of the 30 syntheses of evidence involved 63 external and independent expert reviewers. Each question had a minimum of two reviewers, one with Great Barrier Reef-relevant expertise, and a second ‘external’ reviewer (i.e. international or from elsewhere in Australia). Three questions were identified as potentially contentious (Q3.6, Q4.3 and Q4.7), and these questions were assigned three reviewers. Reviewers completed a structured peer review form which included questions about the quality, rigour and content of the synthesis, and provided a recommendation (i.e. accept, minor revisions, major revisions). A Lead and Second Editor endorsed the synthesis once question authors had adequately addressed peer reviewer feedback. Lead and Second Editors were also asked to provide assurance that there was a clear line of sight between the body of evidence and the higher-level Evidence Statement to ensure that all Statements were supported by the evidence base. The Editorial Board then collectively checked the Evidence Statements for use of non-technical language, clarity and for any inconsistencies among questions. Once satisfied with the Evidence Statements, the Editorial Board formally signed off each synthesis of evidence.
The Editorial Board was also responsible for managing the peer review process for the Summary and Conclusions outputs. These two outputs were derived from the evidence base provided by the syntheses of evidence, with the Summary containing theme level insights, and the Conclusions containing high-level conclusions that covered the breadth of the evidence base. Given the diversity of topics that were covered in these two outputs, eminent reviewers were required who could bring considerable expertise and high-level experience to determine whether the products were fit for purpose. Three eminent reviewers were appointed, one with experience of Great Barrier Reef issues, one national and working outside of the Great Barrier Reef, and a third international reviewer with an agricultural background. The Scientific Consensus Statement’s Coordination Team was responsible for addressing the reviews in a similar manner to the synthesis of evidence review process. The Editor-in-Chief and a supporting Editor checked the preliminary revisions, and once satisfied, these documents were shared with the question authors for formal endorsement. Following this step, the Editorial Board met to discuss the eminent review process and formally sign off this stage.
Further information: 2022 Scientific Consensus Statement peer review process
Appraisal of the evidence is an important aspect of the synthesis of evidence as it provides the reader and/or decision-makers with valuable insights about the underlying evidence base.
In the 2022 Scientific Consensus Statement, each synthesis included an appraisal of the evidence, which involved assessment of the relevance (spatial, temporal, and overall relevance to the question being addressed), quantity (is it a well-researched topic or not?), diversity (does it contain a mix of experimental, observational, reviews and modelling studies?) and consistency of the evidence base for answering the question (is there agreement or debate within the scientific literature?). This approach meant that for the first time, the Scientific Consensus Statement was able to formally assess the confidence in the scientific evidence for each question based on the overall relevance and consistency of the evidence base, as per the matrix below.
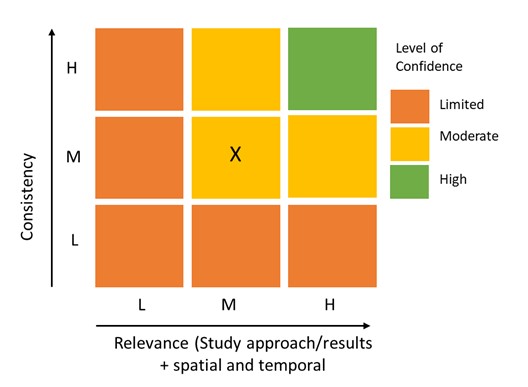
Further information: 2022 Scientific Consensus Statement Methods
There is a strong desire from policy and management representatives involved in Great Barrier Reef water quality management to effectively engage Traditional Owners, and more specifically, involve Traditional Owners to integrate Indigenous people and knowledge into decision-making frameworks for the Great Barrier Reef. Several avenues have been identified to progress this objective, including direct consultation and broader engagement via the update of the Reef 2050 Water Quality Improvement Plan.
After initial consultation with the Great Barrier Reef Foundation’s Reef Trust Partnership Traditional Owner team, and subsequent discussions with policy and management representatives, a question was included in the 2022 Scientific Consensus Statement to review peer reviewed evidence (from Australia and internationally, where relevant) of the success factors for greater Indigenous involvement in water quality decision-making / management that could be applied for the management of the Great Barrier Reef. Question 7.3 (What are the critical success factors for greater Indigenous involvement in water quality decision making in the Great Barrier Reef region?) can be accessed here. However, it was also recognised that alternative sources of evidence (outside of the peer reviewed literature) should be considered in decision-making frameworks for greater Indigenous involvement in Great Barrier Reef water quality management, and also potentially in future Scientific Consensus Statement processes.
Additional effort is required by policy and management to ensure that this is only one step in a larger effort of seeking guidance on the success factors for greater Indigenous involvement in water quality decision-making / management in the Great Barrier Reef. Recognising growing local, national and international discussions about Indigenous rights, free prior and informed consent, partnerships, and Indigenous self-determination, it is recognised that any further work must at a minimum include extensive consultation and input from Traditional Owners.
Further information: Q7.3